Industry Focus: Emerging markets and impacts on water resources
We recently took a look at how rapid growth in demand and a changing energy mix are reshaping the power industry. Some of these forces are also fueling growth in related industries, including the green hydrogen, carbon capture, and energy storage markets, as all of these serve a role in renewable energy. Here we’ll take a look at these key emerging markets, how they’re changing, and what impacts they are likely to have on water resources.
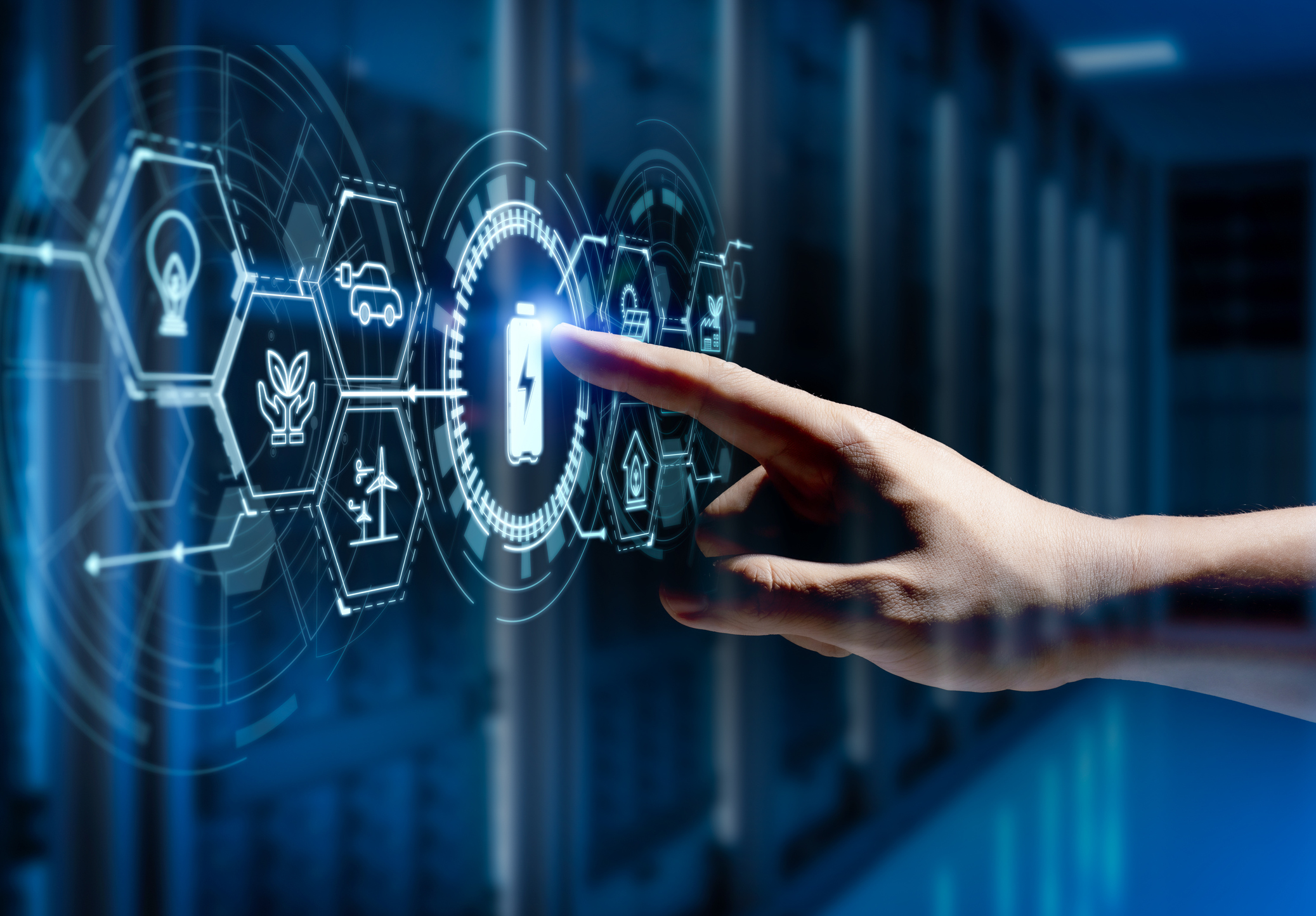
Green hydrogen
Hydrogen offers a promising alternative to fossil fuels because it combusts cleanly without releasing greenhouse gases. The trouble is that the process of making hydrogen fuel can have carbon costs of its own. Most of the hydrogen fuel produced today uses fossil fuels as feedstocks, including natural gas (blue hydrogen), methane (gray hydrogen), and coal (brown hydrogen). In addition, these methods of hydrogen fuel production require significant energy inputs, which only add to the carbon footprint if the energy is derived from fossil fuels.
In contrast, green hydrogen uses renewable energy to power an electrolysis reaction that uses water as a feedstock, making for a low- to zero-carbon process. Unfortunately, green hydrogen is much less common as current technologies make it much more expensive to produce. To put it in perspective, green hydrogen accounted for less than 1% of the 97 megatonnes (Mt) of hydrogen produced across the globe in 2023, according to an International Energy Agency (IEA) report.[1] While figures like this demonstrate that green hydrogen is still very much in its infancy, there are signs that that’s beginning to change. As noted by the IEA, supportive government policy is beginning to drive growth in the green hydrogen market, particularly around electrolysis projects.[2] An example of this is the 45V Clean Hydrogen Production Tax Credit in the US. [3] While this program was first proposed under the Inflation Reduction Act of 2022, it took years of debate and revision before the final rules were released in early January 2025. Under the program, hydrogen producers can claim a tax credit up to $3 per kilogram of clean hydrogen, which can include hydrogen produced by electrolysis, as well as “turquoise” hydrogen, which is produced using renewable natural gas (RNG) derived from derived from landfills, wastewater, animal manure, or other sources of organic waste. In this way, the 45V tax credits aim to subsidize the cost of clean hydrogen production to make it more competitive with fossil fuel-derived hydrogen. It will take some time to see what effect the 45V credits will ultimately have, but many believe that it, along with comparable policies throughout Europe and Asia, will drive accelerated growth and innovation in the clean hydrogen market in the months and years to come.
How growth in green hydrogen might impact water resources
The main method of producing green hydrogen is electrolysis, a process that uses water as a feedstock. For this reason, there is some concern that a growing green hydrogen market would mean net increases in water use. According to a Deloitte analysis of various studies on the topic, while green hydrogen produced using renewable energy sources has a lower water footprint than other hydrogen production methods, the water footprint for electrolysis is still comparable with that of natural gas extraction and processing.[4] So while green hydrogen might be good for lowering carbon emissions, in its current state, the technology does little to curb water use in fuel production. There is hope, however, that further research and development will yield advancements that allow for more efficient use of water and energy resources in the production of green hydrogen.
Clean hydrogen incentives may also impact how communities and industrial facilities choose to treat and dispose of wastewater. In allowing for the use of RNG as a feedstock for clean hydrogen production, the Section 45V credit could potentially incentivize facilities to capture and use methane from wastewater or other sources. Such incentives may encourage capital investments into anaerobic digesters or other technologies that allow facilities to capture byproducts of wastewater treatment for clean hydrogen production while also producing additional benefits, like reducing sludge production and energy consumption used in wastewater treatment.
Carbon capture
Carbon sequestration involves a few different technologies, all of which are focused on mitigating greenhouse gas emissions. Carbon capture utilization and storage (CCUS) is a term that broadly encompasses multiple technologies that remove CO2 from emissions at the source– think smokestacks outfitted with scrubbers or other such technologies. These technologies can be further distinguished by the ultimate destination of the captured CO2. In carbon capture and storage (CCS), the CO2 is compressed and transported for permanent underground storage, while in carbon capture and utilization (CCU), the CO2 is repurposed for other uses, such as enhanced oil recovery (EOR), or production of synthetic fuel or fertilizer. In addition to these, there are also carbon removal technologies, which are designed to remove CO2 that has already entered the environment from past emissions. This can include direct air and marine carbon removal.
As many work to meet emissions goals, CCUS projects have seen a growing interest. The Global CCS Institute reported that global CO2 capture capacity totaled 51 million tonnes per annum (Mtpa) globally as of the close of 2024, with an additional 51 Mtpa under active construction.[5] Further, a recent IEA report projected that carbon storage capacity will grow to 430 million metric tons by 2030.[6]
Despite these growth patterns, the pace of implementation is still falling far short of the rate needed to meet net-zero emissions commitments. As ING analysts note, carbon storage projects are often hampered by high capital costs, uncertainties over environmental policy, and lack of infrastructure for transporting CO2, both in the US and abroad.[7] Similarly, a recent McKinsey analysis suggested that the growth in CCUS projects might also be inhibited by opposition from local governments and communities as well as potential slumps in demand as green hydrogen becomes more cost-competitive with blue hydrogen.[8] At the root of some of these limiting factors is debate over the environmental impact of carbon storage, as well as the overall efficacy of capture and removal as tools for addressing carbon emissions. For example, a study published in February 2025 by civil and environmental engineering researchers at Stanford University found that an overall cost-benefit analysis strongly favors the use of clean renewables over the use of carbon capture to simply mitigate emissions.[9] In the study, the researchers went as far as to suggest that carbon capture should be abandoned entirely, and that resources should instead be used to expand non-combustion energy generation, such as wind, solar, geothermal, and hydropower.[10]
Perhaps because of such debates, the use of CCUS has largely been confined to industries that struggle to manage carbon emissions through other means, such as the cement, petrochemical, and refinery industries.[11] But there is evidence that this is changing. The IEA reports that 2024 saw expansion of CCUS projects into new geographical regions and new industrial sectors, such as natural gas power plants,[12] suggesting that debates around carbon sequestration and what role it should play in sustainability strategies are far from settled.
How growth in carbon capture might impact water resources
The water footprint of CCUS technologies is surprisingly large. Facilities may use water for cooling, as well as formulating solvents and adsorbent regeneration solutions used in carbon capture equipment. While these direct uses account for a portion of the CCUS water footprint, a much larger share can be attributed to all the energy required to separate, compress, and transport CO2. In fact, for facilities that derive some or all of their energy from fossil fuels, carbon capture may yield little to no net reduction in carbon emissions, while consuming large volumes of water in the process. As a Deloitte analysis shows, increased use of clean energy sources as well as the use of more efficient energy generation and cooling technologies have allowed power plants to cut their water use, but increasing use of CCS technologies threatens to undo some of that progress.[13] In short, if CCUS is to play a role in long-term environmental sustainability projects, then further advancements will be needed to improve their water and energy efficiency.
Energy storage
Energy storage is a means of storing excess energy to provide a consistent power supply during periods of lower production or high demand. The most common examples of energy storage devices include pumped-storage hydropower and batteries. For the past several years, pumped-storage hydropower has served a valuable role in grid-scale energy storage, hovering around 23 GW of electric generating capacity in the US.[14] It is an efficient and renewable method of energy storage that can be used to enhance grid stability, but its capital costs run high, and it can only be used in regions with suitable geography.
Batteries represent another key sector in the energy storage market—one that has seen rapid growth in recent years and is closing the gap on pumped-storage hydropower for grid-scale storage.[15] According to US EIA data, battery storage capacity grew from 1 GW to 26 GW from 2020 to 2025. This growth trend is expected to continue, with projections showing that the current battery storage capacity will more than double by the end of 2026.[16] Much of this growth trend can be attributed to the fact that battery technologies have advanced significantly in recent years, allowing them to perform better, longer, and more safely than ever before.[17]
Lithium-ion batteries are particularly dominant, with use applications including electric vehicles (EVs), wind and solar energy storage, and consumer electronics. For this reason, the lithium market is closely entwined with renewable energy and electrification trends. As such, growth in these areas is inspiring strong growth in the lithium market too. In fact, Fortune Business Insights forecasts that the global lithium market will nearly quadruple in size within the next seven years, reaching $55 billion in value by 2032.[18]
How growth in energy storage might impact water resources
There’s no doubt that energy storage is an essential part of a clean energy future. But producing all those batteries takes a lot of water. Battery manufacturers use water for many applications, such as preparing slurries of electrode materials, electrolyte solutions, washing and cleaning, and cooling. But the larger part of the water footprint is higher up in the supply chain, stemming from extraction of minerals such as lithium, cobalt, nickel, aluminum, lead and other minerals used in the manufacture of batteries. Lithium extraction, in particular, is a water-intensive process that involves separating lithium from underground brine deposits or mineral ore. To make matters more challenging, there are relatively few lithium-rich deposits on Earth, and most of them are located in water-stressed regions.
Battery manufacturers have become increasingly conscious of the need to manage water resources well to ensure that they can scale to meet growing demand. So, too, have the lithium producers that supply them, with many recognizing that water pollution and heavy water use may jeopardize the long-term sustainability of extraction operations. According to Fortune Business Insights, mining companies are increasingly investing in research to identify ways to mine lithium more responsibly, and improve recycling methods.[19] Additionally, mining companies are increasingly recycling freshwater, with some achieving a reuse rate of 90%, according to Deloitte.[20] As these trends continue, it is likely that lithium producers will continue to seek out strategies to maximize lithium recovery and use resources more efficiently.
How can SAMCO help?
SAMCO has over 40 years’ experience custom-designing and manufacturing water treatment systems designed to conserve water and energy resources, so please feel free to reach out to us with your questions.
If you’re interested in learning about the best water treatment technologies for green hydrogen, carbon capture, battery manufacturing, lithium extraction, or other specialty applications, we invite you to contact us. Our engineers will walk you through the process of developing a water treatment solution to meet your specific objectives. You can also request a quote to help you develop a realistic budget.
You can also check out our blog to learn more about industrial filtration and process separation technologies. Some articles that might be of interest to you include:
- Water Recycling and Reuse Systems: Strategies for a Smaller Carbon Footprint
- Power Generation and How Water Systems Make or Break It
- Industry Focus: How the Power Industry is Evolving and What it Means for Your Wastewater Management Strategy
- What Is Lithium Extraction and How Does It Work?
- How is Brine Mining Used for Lithium Recovery?
[1] International Energy Agency (IEA), “Global hydrogen review 2024,” Paris. Oct. 2024. Retrieved Apr. 29, 2025.
[2] Ibid.
[3] US Department of the Treasury (USDT), “Credit for production of clean hydrogen and energy credit,” 90 Fed. Reg. 2224. Published Jan. 10, 2025.
[4] K. Hardin and A. Christian, “Can evolving energy technologies help reduce the sector’s impact on water stress?,” Deloitte Insights, Aug. 21, 2024. Retrieved May 1, 2025.
[5] Global CCS Institute, “The Global Status of CCS: 2024,” Australia, Mar. 25, 2025 (pp. 12). Retrieved May 1, 2025.
[6] M. Fajardy, C. Greenfield and J. Tweneboah Koduah, “CCUS projects around the world are reaching new milestones,” IEA, Apr. 30, 2025. Retrieved May 1, 2025.
[7] G. Hieminga and C. Zhang, “Carbon capture and storage: Gaining ground, despite challenges,” ING Think, Jan. 22, 2025. Retrieved May 1, 2025.
[8] K. Biniek, L. Di Fiori, R. Liffey, N. Segel, and B. Stackhouse, “Global energy perspective 2023: CCUS outlook,” McKinsey Energy Solutions, Jan. 24, 2024. Retrieved May 1, 2025.
[9] M. Z. Jacobson, D. Fu, D. J. Sambor, and A. Mühlbauer, “Energy, health, and climate costs of carbon-capture and direct-air-capture versus 100%-wind-water-solar climate policies in 149 countries,” Environmental Science & Technology 2025 59 (6), 3034-3045, Feb. 9, 2025. Retrieved May 1, 2025.
[10] Ibid.
[11] G. Hieminga and C. Zhang, “Carbon capture and storage: Gaining ground, despite challenges,” ING Think, Jan. 22, 2025. Retrieved May 1, 2025.
[12] M. Fajardy, C. Greenfield and J. Tweneboah Koduah, “CCUS projects around the world are reaching new milestones,” IEA, Apr. 30, 2025. Retrieved May 1, 2025.
[13] K. Hardin and A. Christian, “Can evolving energy technologies help reduce the sector’s impact on water stress?,” Deloitte Insights, Aug. 21, 2024. Retrieved May 1, 2025.
[14] US Energy Information Administration (EIA), “Short-term energy outlook data browser table 7e. US electric generating capacity,” Apr. 10, 2025. Retrieved Apr. 29, 2025.
[15] IEA, “Grid-scale storage,” Jul. 11, 2023. Retrieved May 1, 2025.
[16] EIA, “Short-term energy outlook data browser table 7e. US electric generating capacity,” Apr. 10, 2025. Retrieved Apr. 29, 2025.
[17] Fortune Business Insights, “Stationary energy storage market size: Global report [2032]”,” Apr. 14, 2025. Retrieved May 1, 2025.
[18] Fortune Business Insights, “Lithium market size, share and trends: Growth forecast [2032],” Apr. 14, 2025. Retrieved May 1, 2025.
[19] Ibid.
[20] K. Hardin and A. Christian, “Can evolving energy technologies help reduce the sector’s impact on water stress?,” Deloitte Insights, Aug. 21, 2024. Retrieved May 1, 2025.